
Tomorrow’s trucks, trains, ships, and airplanes could be powered with clean hydrogen technology that exists today—and discoveries made by Canadian physicists could help make this sustainable technology even safer and more efficient.
Source: Canadian Neutron Beam Centre (CNBC)
Contact: cnbc@cnl.ca
Image: Toyota has already demonstrated hydrogen-powered transport trucks. (Toyota.com)
Hydrogen technology has long been considered an important component of any clean energy future due to its sustainability and its low impact on the environment. Indeed, when hydrogen is burned as fuel, its only by-product is water, which can simply be converted back into hydrogen.
Soon, nobody will be able to imagine life without hydrogen technology. – Takeshi Uchiyamada, Chairman of Toyota Motor Corporation
Proponents of hydrogen technology judge it to be particularly useful in terms of enabling the widespread integration of renewable energy sources. For instance, hydrogen has the potential to smooth out seasonal fluctuations in energy supply, such as by storing some of the solar energy produced in the summer months for use in the winter. Hydrogen can also be substituted for fossil fuels in industrial applications that are hard to electrify, and it can be easily transported to locations that are not connected to the electricity grid.
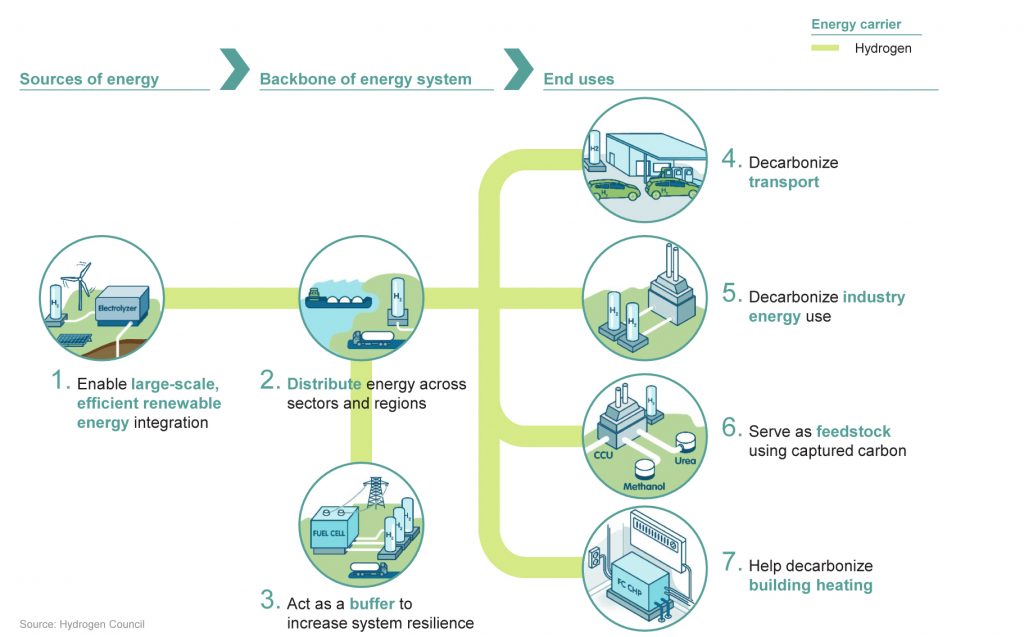
Hydrogen technology can enable the transition to a clean energy future by displacing fossil fuels in seven major areas of our economy, including examples mentioned in the main text: smooth out seasonal fluctuations in energy supply (1), transporting energy to locations not connected by a grid (2), substituting for fossil fuels in transportation (4) and industrial applications (5). (Image: Hydrogen Council)
For reasons such as these, industry leaders like Takeshi Uchiyamada, Chairman of Toyota Motor Corporation, are among the most vocal champions of this technology. “Hydrogen technology has the widespread support it needs to scale up,” affirmed Uchiyamada while speaking about hydrogen’s role in a sustainable economy. “Soon, nobody will be able to imagine life without it.”
Toyota is part of the newly formed Hydrogen Council, a 24-member industry consortium launched in 2017 that will invest more than $10 billion over the next 5 years in the development and deployment of hydrogen technology. Toyota’s participation in the consortium is not surprising, as the auto industry is particularly interested in deploying hydrogen technology as a means of decarbonizing the transportation sector.
When it comes to reducing the emissions linked to transportation, batteries (e.g., lithium–ion batteries) have had an early lead in the electric car market. However, hydrogen fuel cells hold greater promise for larger vehicles, such as transport trucks. That’s because the batteries needed to power a fully-loaded transport over a long distance would take up over 50 percent of the truck’s legally allowed weight limit, leaving less than half of its capacity for goods.
There is need for researchers to perfect how hydrogen is stored for use in transport applications.
Toyota has demonstrated a tractor-trailer that solves this weight problem by using a hydrogen fuel cell to recharge its single battery en route. However, these trucks—which have been transporting containers to and from two busy California ports since October 2017—run only short routes to nearby warehouses and railyards because their refuelling ability is largely limited by the availability of hydrogen fueling stations.
Toyota is not alone in its quest to get hydrogen-powered trucks on the road. Nikola Motor Company has recently unveiled an 18-wheel hydrogen-powered transport with a range of nearly 2000 kilometres—and the company has ambitious plans to build hundreds of fueling stations across the U.S.
Not surprisingly, there is need for researchers to perfect how hydrogen is stored for use in transport applications. Today, hydrogen-powered vehicles store their hydrogen in highly pressurized tanks. While these tanks have been shown to be just as safe as gasoline tanks, the research community sees an opportunity to completely eliminate any risk of fire or explosion by storing the hydrogen within a solid material instead. If realized, this approach would reduce the need to pressurize the hydrogen and would also minimize the space taken up by the hydrogen storage system in the vehicle—all while potentially reducing the cost of hydrogen-powered transportation.
Jacques Huot, a professor of physics at Université du Québec à Trois-Rivières, is one researcher who is anxious to learn more about the materials that could potentially make solid hydrogen storage a reality. Huot collaborates with Julien Lang, a Research Scientist with Canadian Nuclear Laboratories (CNL). Together, these researchers collaborate to investigate metal hydrides—a class of materials with good potential to meet the performance standards for hydrogen storage systems set out by the U.S. Department of Energy.
Metal hydrides are a class of materials with good potential to meet the performance standards for hydrogen storage systems set out by the U.S. Department of Energy.
Specifically, Huot and Lang are studying an alloy known as magnesium iron hexahydride (Mg2FeH6), which has the highest hydrogen density by volume of any known material—even higher than pure hydrogen! In fact, a tank filled with pure liquid hydrogen (which requires cooling to –253 °C) would contain only half the number of hydrogen atoms as the same volume of solid Mg2FeH6. This high hydrogen density makes scientists hopeful that Mg2FeH6 might be used to enable transport vehicles to store sufficient fuel for long-range trips.
Numerous researchers have sought to understand the chemical processes involved in the absorption and release of hydrogen in metal hydrides such as Mg2FeH6. However, this line of research is rife with challenges. For one thing, it is difficult to make Mg2FeH6 because magnesium and iron are like oil and water—they don’t mix well. In addition, it can be difficult to determine exactly what role the hydrogen is playing during a chemical process because hydrogen atoms are the smallest of all atoms, so they can easily remain undetected by x‑rays and most other scientific probes when surrounded by larger metal atoms.
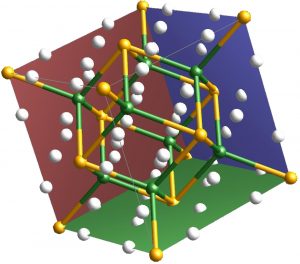
Illustration of Mg2FeH6, composed of iron (green circles), magnesium (yellow), and hydrogen (white) atoms. (Image: Lang)
Huot had previously studied various approaches for synthesizing Mg2FeH6, each of which produces varying results in terms of both the efficiency of the Mg2FeH6 formation process as well as the material’s subsequent absorption and release properties. Since the key to designing an effective Mg2FeH6 hydrogen storage system may be hidden in the chemical processes at work during both the synthesizing and the later absorption and release stages, Huot needed to find a way to elucidate these processes.
To help him with this task he turned to Julien Lang, who was then a post-doctoral researcher working under the guidance of CNL scientist Helmut Fritzsche. Lang specializes in using neutron beams to understand how materials absorb and release hydrogen atoms. Neutron beams are excellent for investigating the role of hydrogen in materials because neutrons are able to detect atoms of varying sizes, no matter how small. What’s more, it is possible to enhance the contrast between the hydrogen and the surrounding atoms by exchanging normal hydrogen with deuterium, a form of hydrogen that differs only by the presence of a neutron in the atomic nucleus. In this way, the hydrogen atoms become even more visible to the neutron beams.
Neutron beams are excellent for investigating the role of hydrogen in materials because neutrons are able to detect atoms of varying sizes, no matter how small
Lang used the Canadian Neutron Beam Centre to conduct a series of experiments (some using normal hydrogen, some using deuterium, and some using a mixture of the two) to identify the signatures of the hydrogen within the material at various stages of Mg2FeH6 formation. Importantly, the neutron data enabled Lang and Huot to determine what phases (i.e., the spatial patterns of the magnesium, iron, and hydrogen atoms) were present at each stage during the Mg2FeH6 formation process.
The findings confirmed a hypothesis that arose from Huot’s earlier research, which hinted at the mechanism by which Mg2FeH6 forms. The results also showed that the iron acts as a catalyst to separate hydrogen atoms from each other (normally, hydrogen gas takes the form of molecules composed of two hydrogen atoms bound together). These findings were published in 2017 (doi:10.1016/j.ijhydene.2016.11.157).
Interestingly, some unexpected findings from the neutron beam experiments revealed further complexities to Mg2FeH6 that are still not understood. For instance, the researchers observed the presence of a special iron phase, which seems to be a by-product of Mg2FeH6 formation. Usually, this iron phase is formed only at temperatures exceeding 900 °C—and yet it was detected during the room-temperature synthesis of Mg2FeH6.
Today, Huot and Lang, are continuing their collaboration to further unravel the complexities of Mg2FeH6 and other metal hydrides. In the end, even if Mg2FeH6 turns out to be unsuitable for hydrogen storage, the insights they are gaining through their neutron beam investigations could lead them to identify another metal hydride that has the ideal characteristics to form the basis of tomorrow’s safe and sustainable hydrogen-fuelled transportation systems.